Joo group has laid a foundation on the utilization of gas-assisted electrospinning and air-controlled electrospray in the development of nanomaterials for energy storage devices.
Fig. 1 Schematics of gas-assisted electrospinning and air-controlled electrospray processes for controlling the nano-scale assembly in energy storage materials
Nanofibers directly deposited electrodes via scalable gas-assisted electrospinning and air-controlled electrospray are able to overcome many issues in energy storage materials for the following reasons:
♦ Low cost, high throughput manufacturing based on gas-assisted and water-based electrospinning and air-controlled electrospray
♦ Nonwoven nanofiber mat control:
– Shape and porosity control
– Highly conductive interconnected network
– Mitigation of volume expansion of Si via pores among fibers
– High porosity and ionic conductivity for separator
♦ Compositional control within each nanofiber:
– Excellent dispersion control and uniformity
– Consistent performance
♦ Flexibility of material morphology allows for many form factors of battery (button, coin, cylindrical, pouch, and prismatic cells)
The current research focuses on i) Si/graphene hybdrids for Li-ion battery anode, ii) polymer/ceramic hybrids for LIB separator, iii) highly loaded sulfur cathode and coated separator for Li-sulfur batteries, and iv) Metal oxide nanofibers for Li-air battery cathode.
1. Directly Deposited Si/graphene hybrids for LIB Anode
- Using scalable water-based, gas-assisted electrospinning and cir-controlled electrospray processes, we have developed directly deposited high capacity anode materials based on Si/graphene for Li-ion batteries.
- The resulting Li-ion battery anode exhibits high capacity and improved cyclability.
Figure 2. Top: a) Schematic of fabrication of directly deposited Si/CNT/PVA nanofiber anodes and the cyclic performance of the resulting Si/CNT/PVA nanofiber anode, Bottom: b) schematic of fabrication of directed deposited Si/graphene hybrid anode via air-controlled electrospray
2. Nanofiber-based Separator for LIB
- Novel separators based on polymer-ceramic hybrid nanofibers have been developed for high capacity Li-ion batteries.
- Polymer-ceramic separators demonstrated much improved rate capability compared to the polymer (PAN, PI, m-aramid) nanofiber separator and commercial Celgard separator, suggesting that the increased ionic conductivity and wetting behavior by both the ceramic within the membrane and on the surface of the membrane are closely correlated to an increase in rate capability than the porosity (see Fig. 3 a).
- Polymer-ceramic hybrid separator with an interconnected ceramic domain showed superior mechanical and electrochemical properties. The interconnected ceramic domains also affect the polymers ability to crystallize, leading to an increase in amorphous regions. The increase in amorphous regions leads to increased electrolyte uptake, superior ionic conductivity and excellent rate capability. The surface morphology covered with ceramic layer was kept constant for all three hybrids (see Fig. 3 b).
Full Cell Performance of Nanofiber-Based Li-ion Batteries
- The full battery cell with Si rich carbon nanofiber-based anode/nanofiber separator/Li metal oxide cathode exhibits the initial energy density over 650 Wh/kg, and around 600 Wh/kg after 25 cycles.
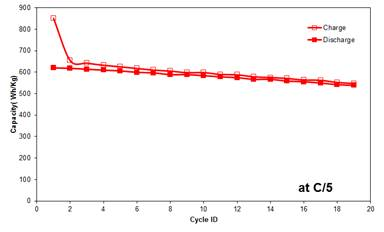
3. Highly Loaded Sulfur Cathode and Coated Separators for Li-S Batteries
- We synthesize mesoporous carbon nanofiber webs (MPCNFWs) via gas-assisted electrospinning PAN/PMMA blend.
- High loading of sulfur (3.5 – 6.2 mg/cm2) is incorporated into MPCNFW via air-controlled electrospray.
- Li-S coin cells with MPCNFW cathode and interlayer exhibits great rate capability with capacity of 900 mA h g-1 at 2C.
- Li-S coin cells based on MPCNFWs lead to an areal capacity of 6.2 mAh cm-2 after 100 cycles at C/4.
- Li-S pouch cell (2” x 3”) with 5.5 mg cm-2 of S loading exhibit 845 mAh g-1 after 100 cycles at C/4.
- PEDOT:PSS and rGO have been coated on the surface of a separator using air-controlled electrospray.
- The capacity and rate capacity are drastically improved via coating the separator, lead to more than twice the capacity, over 800 mAh/g after 100 cycles, at 0.5C rate when it is compared to those with the pristine separator.
Figure 5 Schematic of fabrication of highly loaded sulfur cathode and its performance
Figure 6 Schematic of coating the separator with PEDOT:PSS and rGO, and its performance
4. Nanostructured Hybrids for Li-O2 Batteries
- Hybrids of nanostructured carbon and metal oxide nanofibers have been devised as a cathode material for Li-air batteries, and inclusion of metal oxide nanofibers and the presence of mesopores significantly increases the capacity of Li-air batteries (see Fig. 7).
- The increase in cyclability by utilizing Pt/carbon mixed with ZnO nanofibers has been demonstrated.
- Disruption of physical structure in the Li-air cathode after cycles is observed, and two-thirds of the initial capacity can be recovered by compacting graphene layers.
- Rearranging the cathode structure help to recover the capacity in the next cycle (see Fig. 8).
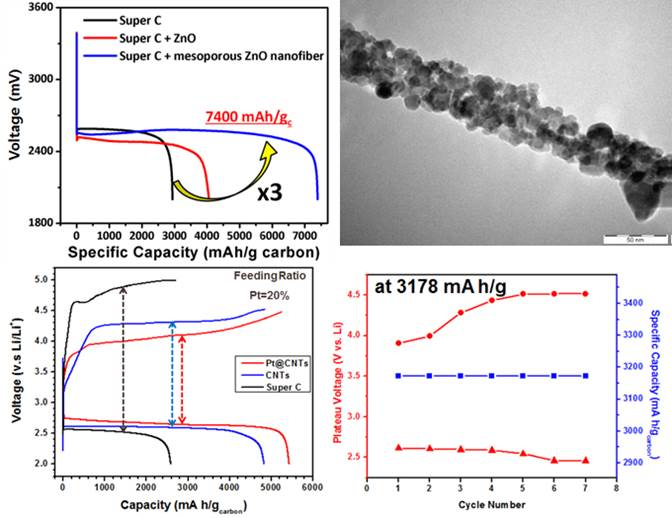
Figure 8. Concept of thermal swing restacking (TSR) (top), and SEM images of RGO cathode before and after TSR (bottom), and enhanced capacity and cyclabliity of Li-air batteries by TSR (right).
Related Publications
- N.W. Utomo, S. Hong, R. Sinha, K. Kim, Y. Deng, P. Ochonma, M.G. Kitahata, R. Garcia-Mendez, Y.L. Joo, and L.A. Archer”Solid-state polymer-particle hybrid electrolytes: Structure and electrochemical properties”, Science Advances 10, eado4719 (2024).
- Chen, S. Jin, S. Hong, Y. Qiu, Z. Zhang, Y. Xu, Y.L. Joo, L.A. Archer, and R. Yang, “Adaptive ion channels formed in ultra-thin and semi-crystalline polymer interphases for stable aqueous batteries”, J. of Amer. Chem. Soc. 146, 3136 (2024).
- Jin, X. Gao, S. Hong, Y. Deng, P. Chen, R. Yang, Y.L. Joo, L.A. Archer, “Fast-charge, long-duration storage in lithium batteries”, Joule 8, 1-18 (2024).
- S. Jin, S. Hong, X. Gao, Y. Deng, Y.L. Joo, L.A. Archer, “Self-sufficient metal-air battery systems enabled by solid-ion conductive interphases”, Faraday Discussions 248, 305 (2024).
- X. Gao, C. Zheng, Y. Shao, V.R. Shah, J. Suntivich, and Y.L. Joo, “ Lithium Iron Phosphate Enhances Performance of High-Areal-Capacity Sulfur Composite Cathodes”, ACS-Applied Materials and Interfaces 15, 19011 (2023).
- S. Jin, P. Chen, Y. Qiu, Z. Zhang, S. Hong, Y.L. Joo, R. Yang, L.A. Archer, “Zwitterionic polymer gradient interphases for reversible Zinc electrochemistry in aqueous alkaline electrolytes”, J. of Amer. Chem. Soc. 144, 19344 (2022).
- S. Jin, Y. Shao, X. Gao, P. Chen, J. Zheng, J. Yin, Y.L. Joo, L.A. Archer “Designing interphases for practical aqueous Zinc flow battery with high power density and high areal capacity”, Science Advances 8, eabq4456 (2022).
- S. Jin, J. Yin, X. Gao, A. Sharma, P. Chen, Q. Zhao, J. Zheng, Y. Deng , Y.L. Joo, L.A. Archer, “Production of fast-charge Zn-based aqueous batteries via interfacial adsorption of ion-oligomer complexes”, Nature Communications 13, 2283 (2022).
- S. Sarkar, J.S. Won, M. An, R. Zhang, J.H. Lee, S.G. Lee and Y.L. Joo, “Tailoring Mesopores and Nitrogen Groups of Carbon Nano-fibers for Polysulfide Entrapment in Lithium-Sulfur Batteries”, Polymers 14, 1342 (2022).
- Y. Joshi, A. Umasankaran, C. Klaassen, M. AlAmer, and Y.L. Joo, “Critical Roles of Reduced Graphene Oxide in the Electrochemical Performance of Silicon/Reduced Graphene Oxide Hybrids for High Rate Capable Lithium-ion Battery Anodes”, Electrochimca Acta 404, 139753 (2022).
- Y. Joshi, S. Zamani, C. Klaassen, and Y.L. Joo,” Mitigation and In-situ Probing of Volume Expansion in Silicon Graphene Hybrid Anodes for High Capacity, High Rate Capable Lithium Ion Batteries”, Advanced Energy and Sustainable Research 2, 2100125 (2021).
- G.L. Shebert, S. Zamani, C. Yi, and Y.L. Joo, “Polysulfide Entrapment and Retardation in Gel Electrolyte Li-S Batteries: Experiments and Modeling”, Journal of Materials Chemistry A 8, 4341 – 4353 (2020).
- M.J. Divvela, R. Zhang, Y. Zhmayev, S. Pinge, J.H. Lee, S.W. Kim and Y.L. Joo, “Control of Formation of Viscoelastic Droplets and Distribution of Nano-inclusions in Functional Deposition for Lithium-Sulfur Batteries”, Soft Matter 15, 6485-6494 (2019).
- B.P. Williams, G.L. Shebert, and Y.L. Joo, “Metal Oxide Coatings on Carbon Electrodes with Large Mesopores for Deeply Charged Zinc Bromine Redox Flow Batteries”, Journal of Electrochemical Society 166, A2245-A2254 (2019).
- A.B. Shah, Y. Wu, and Y.L. Joo, “Direct Addition of Sulfur and Nitrogen Functional Groups to Graphite Felt Electrodes for Improving All-Vanadium Redox Flow Battery Performance”, Electrochimica Acta 297, 905-915 (2019).
- M. AlAmer, A.R. Lim, and Y.L. Joo, “Continuous Synthesis of Structurally Uniform Graphene Oxide Materials in a Model Taylor-Couette Flow Reactor”, Ind. Eng. Chem. Res. 58, 1123-1462 (2019).
- W. Halim, J.H. Lee, S.M. Park, R. Zhang, S. Sarkar, T. O’Neil, E. Chiang, and Y.L. Joo, “Directly Deposited Binder Free Sulfur Electrode Enabled by Air-Controlled Electrospray Process”, ACS Applied Energy Materials 2, 678−686 (2019).
- J.H. Lee, J. Kang, S.W. Kim, W. Halim, M.W. Frey, and Y.L. Joo, “Effective Suppression of the Polysulfide Shuttle Effect in Lithium–Sulfur Batteries by Implementing rGO-PEDOT:PSS Coated Separators via Air-Controlled Electrospray”, ACS Omega 3 16465–16471 (2018).
- G. Shoorideh, B. Ko, A. Berry, M.J. Divvela, Y.K. Kim, Z. Li, B. Patel, S. Chakrapani, and Y.L. Joo, “Harvesting Inter and Intra-Conductivity of Graphene Nanoribbons for Directly Deposited, High-Rate Silicon-Based Anode for Li-ion Batteries”, ACS Applied Energy Materials 1, 1106-1115 (2018).
- A.B. Shah, X. Zhou, P. Brezovec, D. Markiewicz, and Y.L. Joo, “Conductive Membrane Coatings for High Rate Vanadium Redox Flow Batteries”, ACS Omega 3, 1856-1863 (2018).
- J. Lee, B. Ko, J. Kang, Y. Chung, Y. Kim, W. Halim, J.H. Lee, and Y.L. Joo, “Facile and Scalable Fabrication of Highly Loaded Sulfur Cathodes and Lithium-Sulfur Pouch Cells via Air-Controlled Electrospray”, Materials Today Energy 6, 255–263 ( 2017).
- L. Fei, S.H. Yoo, R.A. Villamayor, B.P. Williams, S. Gong, S. Park, K. Shin, and Y.L. Joo, “Graphene Oxide Involved Air-controlled Electrospray for Uniform, Fast, Instantly Dry, and Binder-Free Electrode Fabrication”, ACS Applied Materials & Interfaces 9, 9738–9746 (2017).
- S.A. Smith, B.P. Williams, and Y.L. Joo, “Effect of Polymer and Ceramic Morphology on the Material and Electrochemical Properties of Electrospun PAN/Polymer Derived Ceramic Composite Nanofiber Membranes for Lithium Ion Battery Separators”, Journal of Membrane Science 526, 315-322 (2017).
- S.A. Smith, J.H. Park, B.P. Williams, and Y.L. Joo, “Polymer/Ceramic Co-continuous Nanofiber Membranes via Room-Temperature Curable Organopolysilazane for Improved Lithium Ion Battery Performance”, Special Issue on Batteries and Supercapacitors, Journal of Materials Science 52, 3657-3669 (2017).
- Y. Zhmayev, S. Pinge, G. Shoorideh, G.L. Shebert, P. Kaur, H. Liu, and Y.L. Joo, “Controlling Placement of Spherical Nanoparticles in Electrically Driven Polymer Jets and Its Application to Li-ion Battery Anode”, Small 12, 5543–5553 (2016).
- G. Shoorideh, Y.S. Kim, and Y.L. Joo, “Facile, Water-Based, Direct-Deposit Fabrication of Hybrid Silicon Assemblies for Scalable and High-Performance Li-ion Battery Anodes”, Electrochimica Acta 222, 946-955(2016).
- B.P. Williams, and Y.L. Joo, “Tunable Large Mesopores in Carbon Nanofiber Interlayers for High-Rate Lithium Sulfur Batteries”, Journal of The Electrochemical Society 163, A2745-A2756 (2016).
- J. Kim, J.M Carlin, S.A. Smith and Y.L. Joo, “Thermal Restacking of Graphene Structure to Improve Lithium-air Battery Cyclability”, Electrochem. Communication 70, 43-46 (2016).
- L. Fei, B.P. Williams, S.H. Yoo, J. Kim, G. Shoorideh, and Y.L. Joo, “Graphene Folding in Si Rich Carbon Nanofibers for Highly Stable, High Capacity Li-Ion Battery Anodes”, ACS Applied Materials and Interfaces 8, 5243-5250 (2016).
- L. Fei, B.P. Williams, S.H. Yoo, J.M. Carlin, and Y.L. Joo, “A General Approach to Fabricate Free-standing Metal Sulfides@Carbon Nanofiber Network as Lithium Ion Battery Anodes”, Chemical Communication 52, 1501-1504 (2016).
- Y.S. Kim, Y.L. Joo, and Y.J. Kwark, “Highly Stable Silicon–Carbon–Nitrogen Composite Anodes From Silsesquiazane for Rechargeable Lithium-Ion Battery”, Journal of Materials Science and Technology 32, 185-199 (2016)
- Y.S. Kim, G. Shoorideh, Y. Zhamyev, J.H. Lee, Z. Li, B. Patel, S. Chakrapani, J.H. Park, S. Lee, and Y.L. Joo, “Critical Contribution of Unzipped Graphene Nanoribbons to Stable Silicon Rich–Carbon Fiber Anodes for Rechargeable Li–ion Batteries”, Nano Energy 16, 446-457(2015).
- J. Yin, J.M. Carlin, J. Kim, Z. Li, J.H. Park, B. Patel, S. Chakrapani, S. Lee, and Y.L. Joo, “Synergy between Metal Oxide Nanofibers and Graphene Nanoribbons for Rechargeable Lithium-Oxygen Battery Cathodes”, Advanced Energy Materials 5, 1401412 (2015).
- D. Cho, M. Kim, J. Hwang, J.H. Park, Y.L. Joo, and Y. Jeong, “Facile Synthesis of Porous Silicon Nanofibers by Magnesium Reduction for Application in Lithium Ion Batteries”, Nanoscale Research Letters 10, 424 (2015).
- Y.S. Kim, W.B. Kim, and Y.L. Joo, “Further Improvement of Battery Performance via Charge Transfer Enhanced by Solution-Based Antimony Doping into Tin Dioxide Nanofibers”, Journal of Materials Chemistry A 2, 2833-2837 (2014).
- Y.S. Kim, K.W. Kim, D. Cho, N.S. Hansen, J. Lee, and Y.L. Joo, “Silicon-Rich Carbon Hybrid Nanofibers from Water-Based Spinning: The Synergy Between Silicon and Carbon for Li-ion Battery Anode Application”, ChemElectroChem 1, 220-226 (2014).